Our community
Meet the award-winners and projects leaders supported by the Foundation
Search in our community
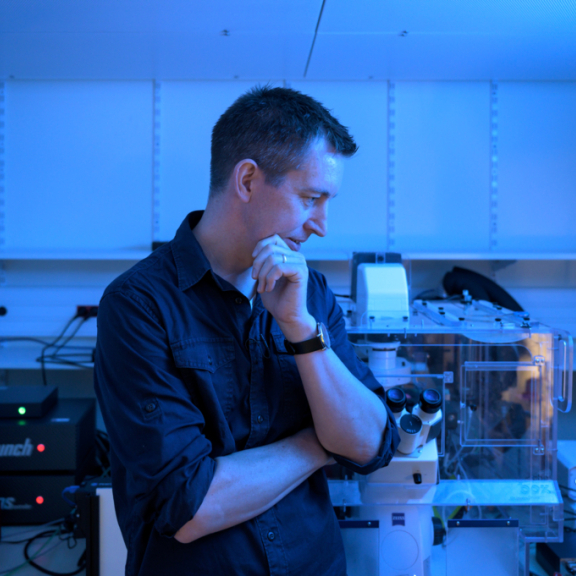
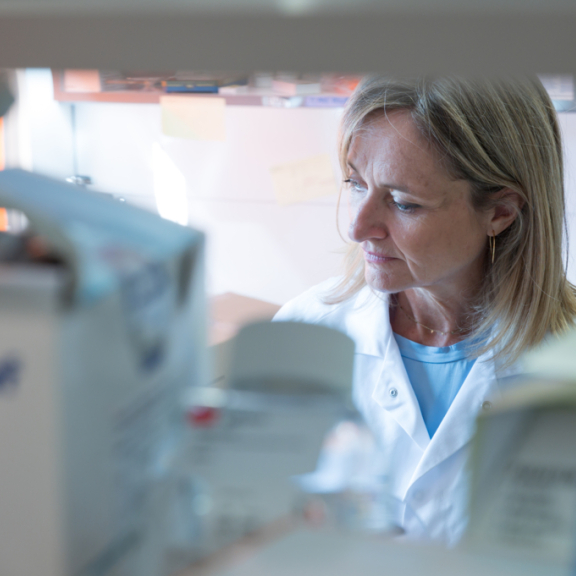
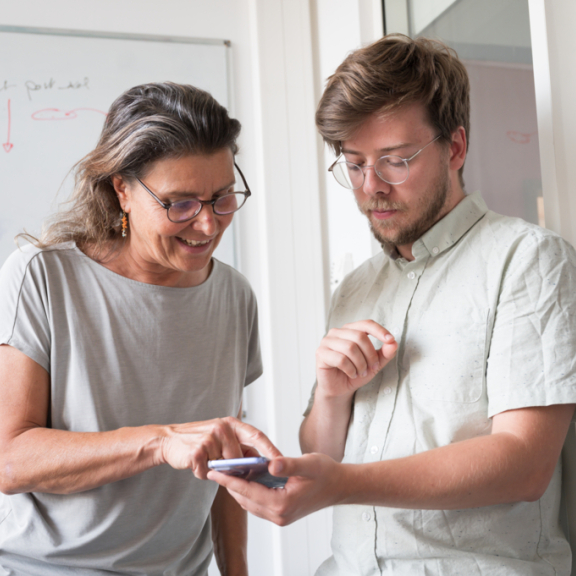
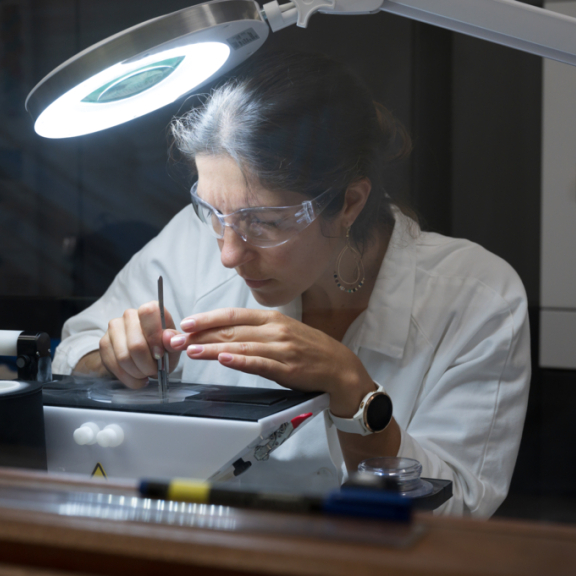
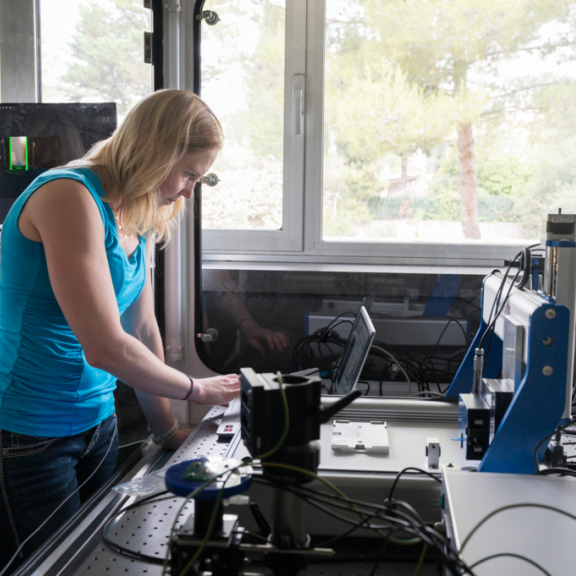
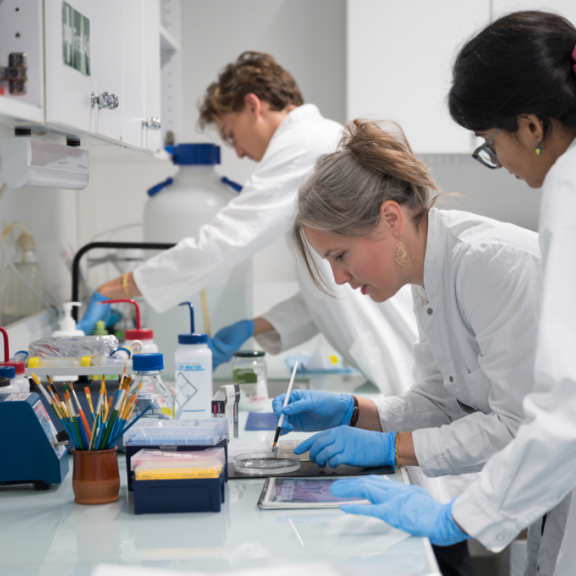
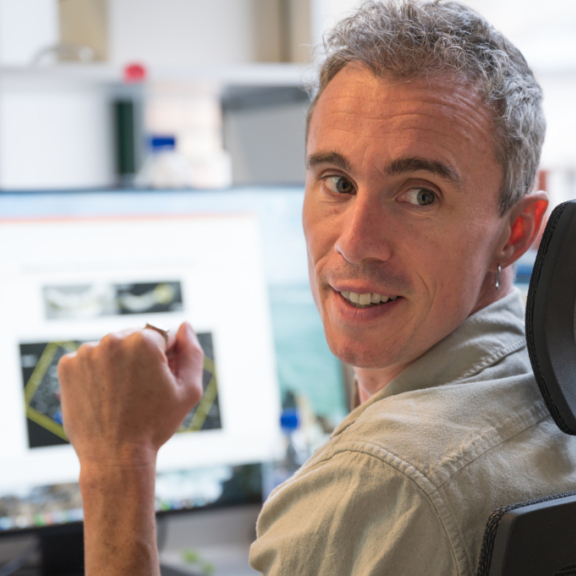
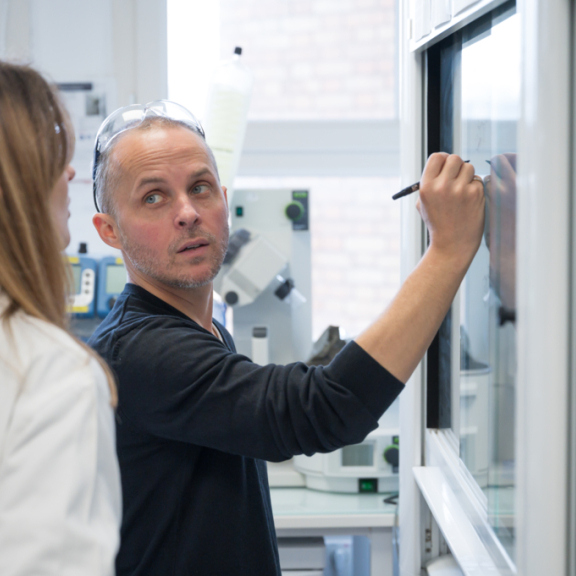
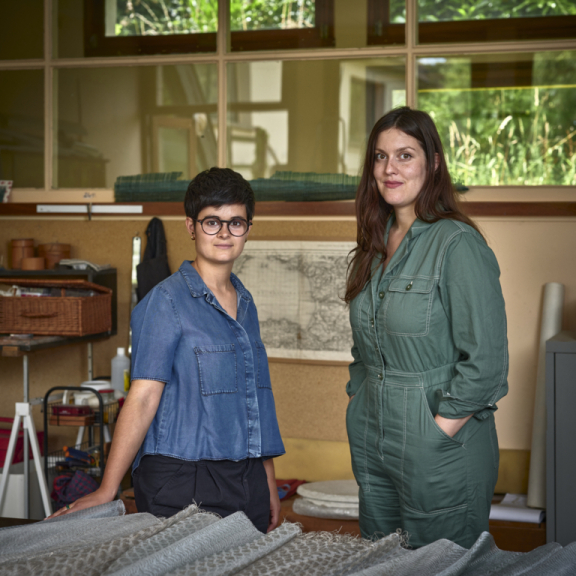
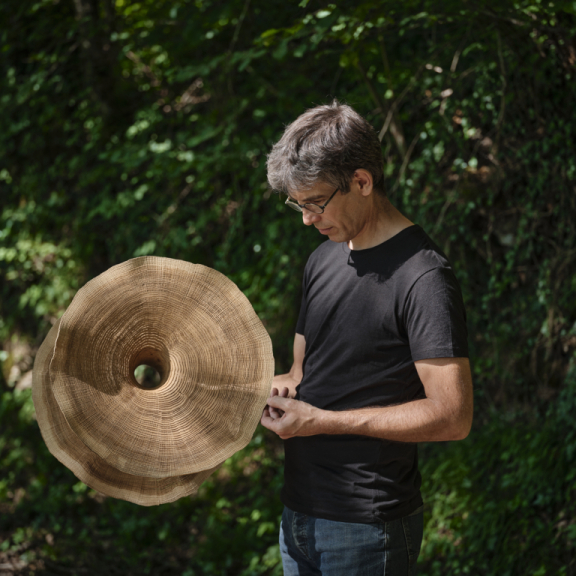
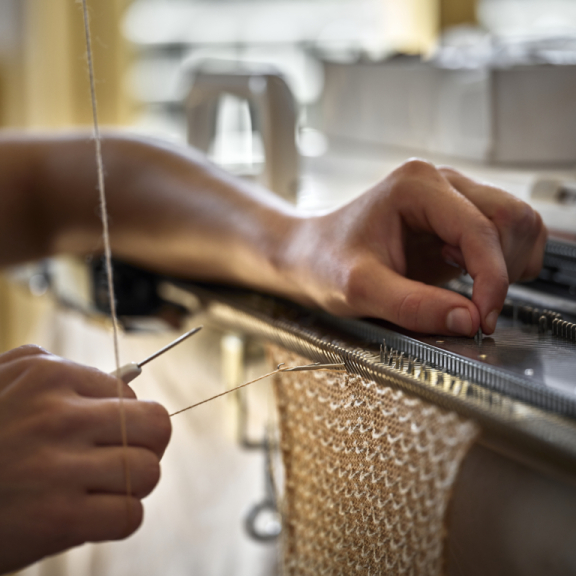
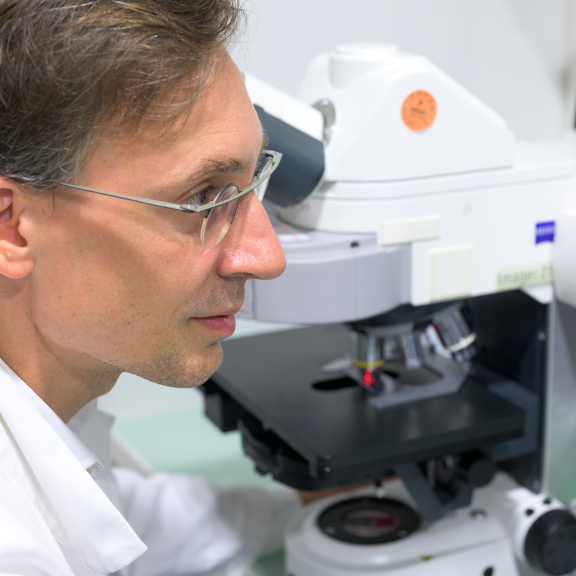
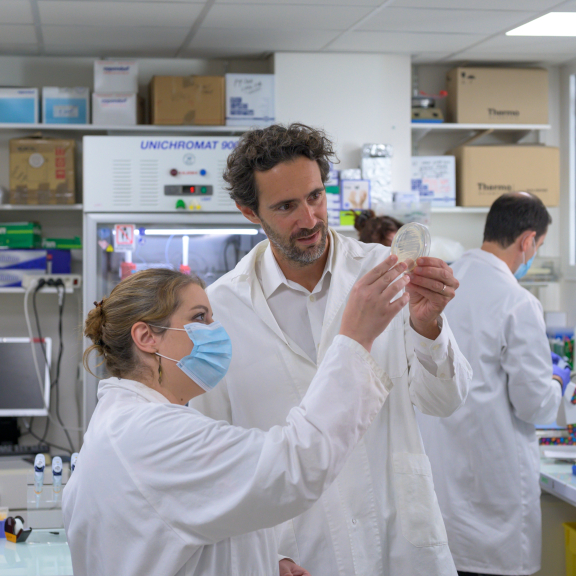
Life Sciences
Antoine Jégou
What are the ingredients needed to build, diversify and maintain the skeleton of a cell?
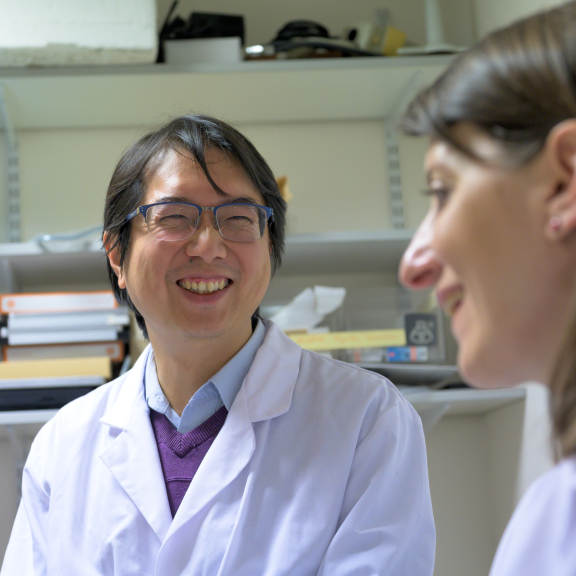
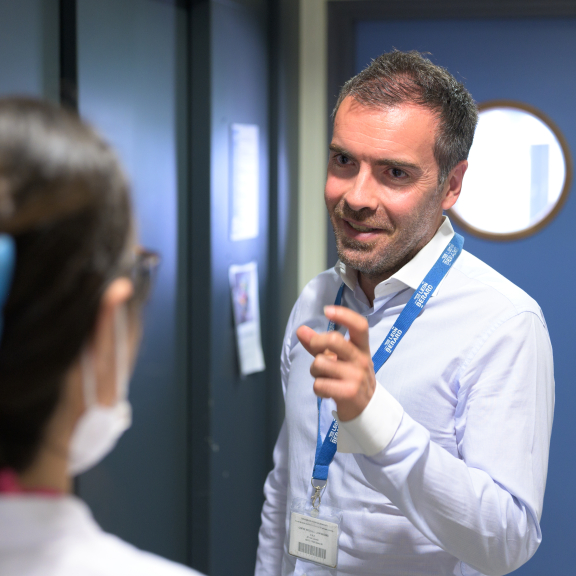
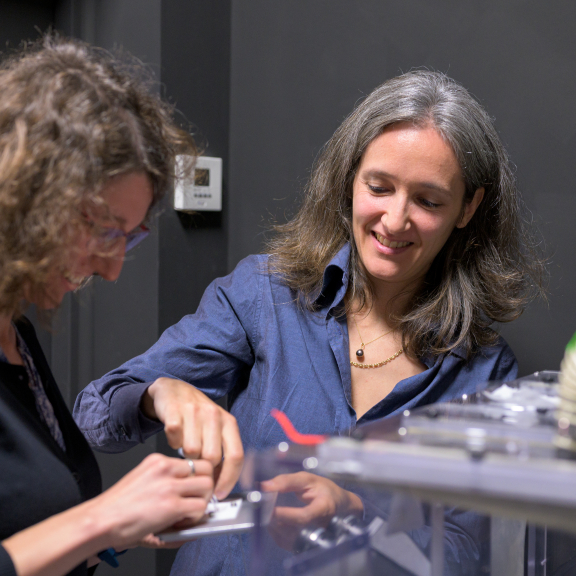
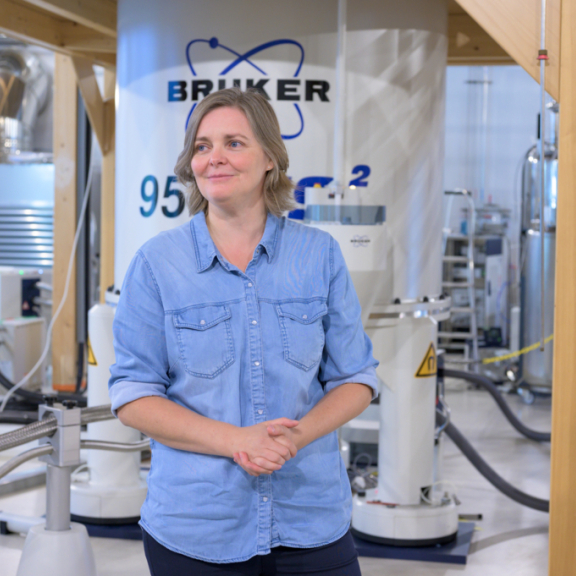
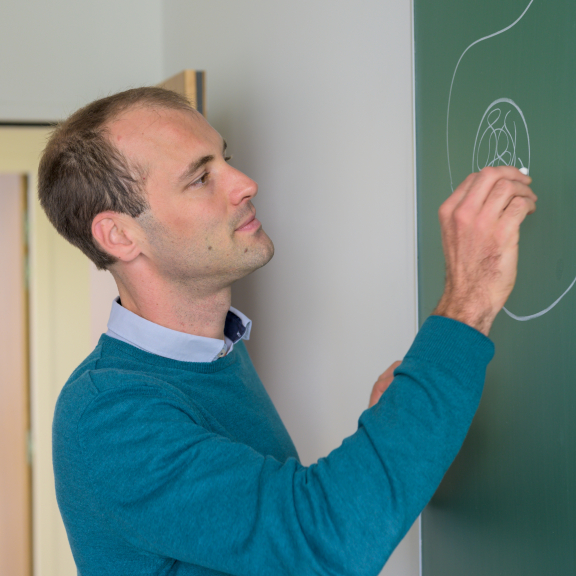
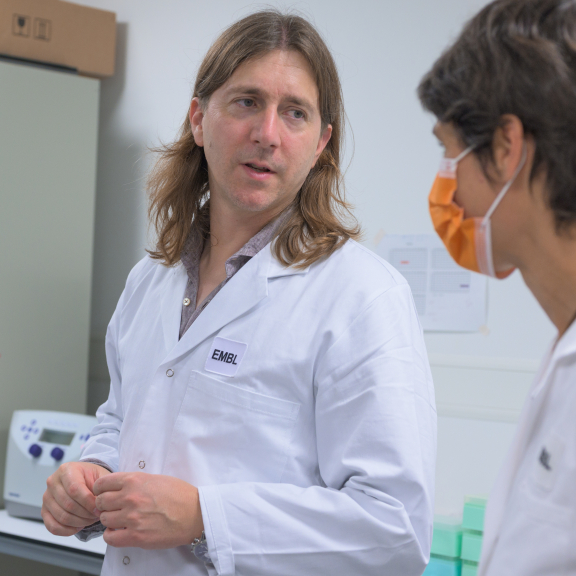
Life Sciences
Athanasios Typas
Creating model bacteria to uncover the hidden treasure of the human gut microbiome